*Guest post by Sheena Fry
Damage caused by agriculture pests is one of the most important
factors of crop yield reduction (Cramer, 1967; Oerke et al., 1994) and can
cause billions of dollars worth of damage each year (e.g. in Brazil, insect
pests cause up to US$ 17.7 billon year-1 of damage, Oliveira et al.,
2014). Due to its economic impact, controlling pest populations is a priority
for agricultural scientists. Chemical control is the primary method of pest
management due to its relatively low costs and high effectiveness (Cooper and
Dobson, 2007). Despite the widespread use of chemical controls, the health and
environmental risks associated with their use are well known (Pimentel et al.
1992; Pimentel, 2005). The risks associated with pesticide use, as well as the
evolution of pesticide resistance, has lead to a surge in interest in the use
of biological control for pest management over the past 50 years.
The most important decision to be made in a biological control
program is which biological control agent to use against a pest. Success rates
for biological control of insects are low, with only 24-35% resulting in the establishment
of the introduced species (Hall and Ehler 1979, van Lentern, 1983) and only 16%
resulting in complete control of pest species (Hall et al., 1980). What
determines the success of colonization and establishment is a key question in
biological control research and must be answered in order to make predictions
about establishment and success of introduced species. In 1965, Debach attempted
to identify characteristics of successful colonizers but found that neither
success nor failure could be explained by the presence or absence of a common
characteristic. Over the past 50 years, several attempts have been made to list
characteristics of successful invaders (e.g. Murdoch et al., 1985) and while
they seem logical, there are too many exceptions for them to be used as a
reliable indicator of a species’ potential to colonize and establish in a new
area. DeBach saw “no possibility of predicting the fate of a purposely
colonized imported entomophagous insect” and at present it remains an elusive
goal (Fischbein and Corley, 2015).
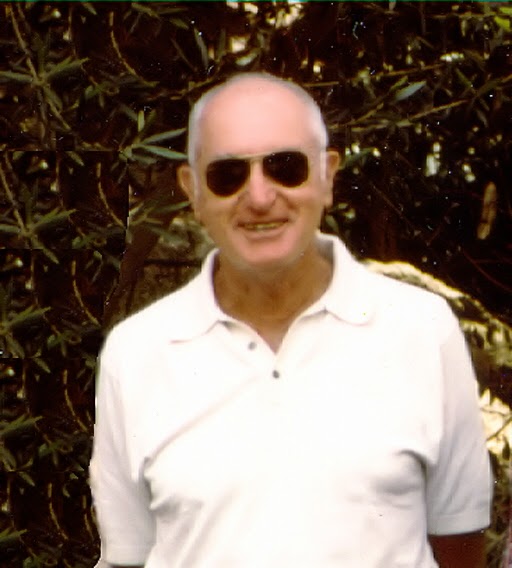 |
Paul Debach 1914-1992 |
The environmental and health risks associated with chemical
controls of insects (see references above) are not an issue when using
biological controls. In addition to this, successfully established biological
control species will be able to maintain stable populations without the need
for additional investment by humans (unlike chemical controls, which must be
applied each season). Despite the obvious benefits of biological control, there
are also risks associated with the use of insects in biological control, such
as the risk to non-targeted species (Simberloff and Stiling, 1996) or host
switching. In order to make decisions about biological control we need to
understand the evolution of introduced species in new environments, which can
increase the efficiency of biological control (through post-colonization
adaptation) or can increase the risk to non-targeted species. “The Genetics of
Colonizing Species” (1965) brought together evolutionary biologists and
ecologists (theoretical and applied) to discuss the evolution of introduced
species. In DeBach’s chapter, he focused on colonizing entomophagous insects
and, using biological control case studies, looked at the relative influence of
pre- and post colonization adaptation, a key question in evolutionary biology. One
such case study was the introduction of a parasitoid wasp (Comperiella bifasciata Howard, Figure 1), which was introduced to
control a citrus pest, the California red scale (Aonidiella aurantii Maskell). The parasitoid wasp was released
throughout southern California but initially was only able to establish at one
location. It slowly spread and increased in abundance and, by 1957 was found at
various locations throughout southern California. DeBach interpreted the poor initial
establishment of the parasite followed by intense colonization as an indication
that genetic adaptation had occurred.
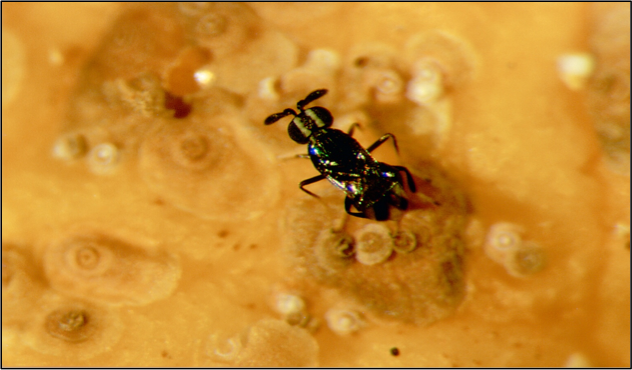 |
Figure 1. A female parasitic wasp (Comperiella bifasciata Howard) infesting
a California red scale (Aonidiella aurantii
Maskell), from Forester et al. (1995).
|
Fifty years have passed since the publication of
“The Genetics of Colonizing Species” (1965) and understanding the relative
effects of pre- and post-colonization adaptation has remained an important
issue. Phillips and colleagues (2008) examined the relative effects of genetic
drift and selection in the frequencies of two asexually reproducing,
genetically distinct parasitoid biotypes. This South American parasitoid wasp (Micrictonus hyperidae Loan, Figure 2)
was introduced as a biological control for a pasture pest (Listronotus bonariensis Kuschel, Figure 2) in New Zealand in 1992.
Phillips and colleagues recorded the relative frequencies of each biotype over
a 10-year period and found that changes in biotype frequency were consistent
with strong directional selection, favouring one of the parasitoid biotypes. This
resulted in parasitoid populations being better adapted to New Zealand
conditions than those originally released.
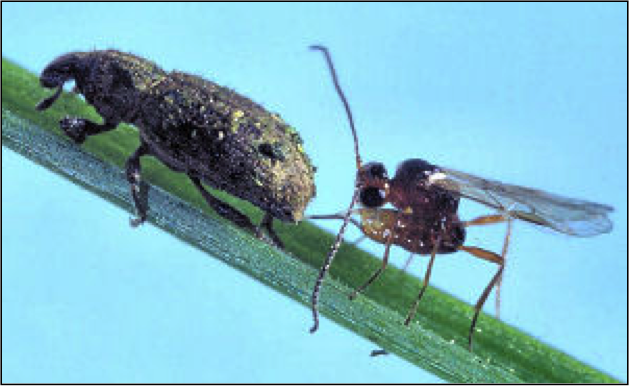 |
Figure 2. A female parasitic wasp (Micrictonus hyperidae Loan, right) infesting a South American
weevil (Listronotus bonariensis
Kuschel, left). © Copyright AgResearch
|
There have been significant advance in the tools (statistical and
molecular) available for the study of post-colonization success and adaptation
since the publication of “The Genetics of Colonizing Species” (1965). These
tools allow for better understanding of the post-colonization process of
introduced species but, despite these advances, there has been little progress
towards being able to predict the success of introduced species.
References:
Baker, H. G., & Stebbins, G.
L. (Eds.). (1965), The Genetics of
Colonizing Species. New
York: Academic Press.
Cooper,
J., & Dobson, H. (2007). The benefits of pesticides to mankind and the
environment. Crop Protection, 26, 1337-1348.
Cramer, H. H. (1967). Plant
protection and world crop production. Pflanzenschutz
Nachr,
20, 1-524.
DeBach, P. (1965). Some
biological and ecological phenomena associated with
colonizing entomophagous insects. In H.G. Baker, and G.L Stebbins
(Eds.).
The Genetics of Colonizing Species. New York: Academic Press.
Fischbein, D., & Corley, J.
C. (2015). Classical biological control of an invasive forest pest:
a worldwide perspective of the management of Sirex noctilio using the parasitoid Ibalia leucospoides (Hymenoptera: Ibaliidae). Bulletin of Entomological Research, 105, 1-12.
Forster, L. D., Luck, R. F.,
& Grafton-Cardwell, E. E. (1995). Life stages of California red
scale and its parasitoids- University of California. Dividion of
Agriculture and Natural Resources. Publication No21529.
Hall, R. W., & Ehler, L. E. (1979).
Rate of establishment of natural enemies in classical
biological control. Bulletin
of the Entomological Society of America, 25, 280-282.
Hall, R. W., Ehler, L. E., and
Bisarbi-Ershadi, B. (1980). Rate of success in classical
biological control of arthropods. Bulletin of the Entomological Society of America, 26, 111-114.
Oerke, E. C., Dehne, H. W.,
Schonbeck, F., & Weber, A. (1994). Crop Production and Crop
Protection- Estimated Losses in Major Food and Cash Crop. Elsevier
Science: Amsterdam.
Oliveira, C. M., Auad, A. M.,
Mendes, S. M., & Frizzas, M. R. (2014). Crop losses and the
economic impact of insect pests on Brazilian agriculture. Crop Protection, 56, 50-54.
Murdoch, W, W., Chesson, J.,
Chesson, P. L. (1985). Biological control in theory and in
practice. The American
Naturalist, 125, 344-366.
Phillips, C. B., Baird, D. B.,
Lline, I. I., McNeill, M. R., Proffitt, J. R., Goldson, S. L., & Kean,
J. M. (2008). Journal of
Applied Ecology, 45, 948-956.
Pimentel, D. (2005).
Environmental and economical costs of the application of pesticides
primarily in the United States. Environment, Development, and Sustainability, 7, 229-252.
Pimentel, D., Acquay, H.,
Biltonen, M., Rice, P., Silva, M., Nelson, J., Lipner, V., Giordano,
S., Horowitz, A., & D’Amore, M. (1992). BioScience, 42, 750-760.
Simberloff, D., & Stiling, P.
(1996). How risky is biological control? Ecology,
77, 1965-
1974.
van Lentern, J. C. (1983). The
potential of entomophagous parasite for pest control.
Agriculture, Ecosystems, and Environment, 10, 143-158.